Hungarian and U.S. Researchers' Discovery Brings Science Closer to Treating Memory Disorders
A groundbreaking discovery by Attila Losonczy and his research group could open new horizons in the treatment of ageing-associated and neurological diseases. The collaborative research conducted by the Zuckerman Institute at Columbia University in New York, the BrainVisionCenter Research Institute and Competence Centre led by Balázs Rózsa, and the HUN-REN Institute of Experimental Medicine has achieved revolutionary results. Using a 3D laser scanning microscope developed by a Hungarian team, researchers succeeded for the first time in observing the formation of memories within living animals in mere fractions of a second, in structures a hundred times thinner than a human hair. The study was published in the prestigious journal Nature.
The recall of memories is based on changes in the strength of connections between brain cells, known as synapses. Although this theory has been around for nearly fifty years, scientists have not been able to directly observe such synaptic changes in living rodent models. Only in recent years have advances in microscopic technologies allowed researchers to study the real-time activity of brain cells in living, behaving animals.
“A deeper understanding of the mechanisms underlying memory formation and consolidation is essential for identifying precise genetic and molecular targets for future therapies,” said Attila Losonczy, Principal Investigator at the Zuckerman Institute at Columbia University, emphasizing the societal benefits of the work. The exploration of these mechanisms is key to the mission of the BrainVisionCenter, founded by Botond Roska and Balázs Rózsa, and the therapeutic and diagnostic mission will be partly carried out at the institute.
The hippocampus, a key region involved in memory, is one of the most studied areas of the brain, but research in recent decades has relied primarily on EEG scans and brain slices. While they are valuable, the inability to study brain processes in real time with high spatial and temporal resolution in living animals limits the capabilities of these methods. Observing neural networks in action is essetnial for a more profound knowledge of brain function, which requires technologies capable of rapidly and accurately scanning cells and synapses within large volumes of tissue.
"Current models of learning and memory are based on the idea that the strength of synapses, or connections between cells in the brain, changes during learning and memory consolidation. Although previous insights into the functioning of synaptic plasticity came from experiments with relatively simple animals, such as sea slugs, or from research conducted under artificial conditions, such as from brain cells grown in laboratories, this model of memory has become extremely successful over the past 50 years and has also served as the basis for the rapid development of artificial intelligence. It is not surprising, however, that studies in live animals have not been done until now, as this has been a huge technical challenge for researchers," explained Attila Losonczy.
The team's research, published in Nature, marks a significant breakthrough in overcoming this obstacle. Their goal was to develop a method to measure long-term synaptic plasticity - the changes in synapse strength that can last for hours or days - in the neurons responsible for learning and memory in real-time, using living rodent models. Achieving this required the specialized two-photon laser scanning microscope developed by the group led by Balázs Rózsa at the HUN-REN Institute of Experimental Medicine and utilized at the BrainVisionCenter. Equipped with 3D real-time motion correction, the system compensates for the brain's constant movement, enabling the study of minuscule brain structures such as cells and their extensions.
“In in-vivo measurements, involuntary movements such as heartbeat and breathing, as well as voluntary movements, can cause displacements of tens of micrometers, much larger than the structures being measured. This, in turn, makes measurements with high spatial and temporal resolution impossible because the biological structures of interest (cell bodies, cell processes, dendrites, dendritic spines) constantly evade the laser scan. The femtosecond [one millionth of a thousand billionth of a second, the time it takes light to travel 0.3 micrometers, roughly the size of a bacterium] laser scanning technique that we use compensates for movement in real time and in 3D," explained Balázs Rózsa, Director of BrainVisionCenter, Principal Investigator at HUN-REN KOKI and co-author of the publication, explaining the usefulness of the new method.
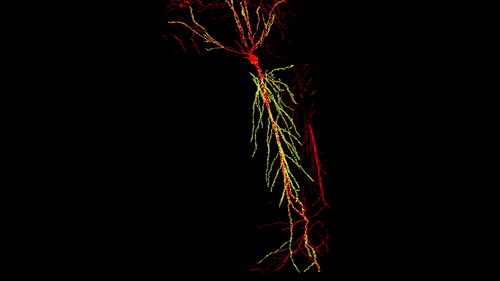
3D reconstruction of a single CA1 pyramidal neuron in the mouse brain. The dendritic arbor is in red and each yellow dot is a mapped excitatory synapse received by this neuron. Each CA1 pyramidal neuron in the mouse receives between 10,000 to 15,000 excitatory synapses.
Photographer: Daniel Iascone | Polleux lab
The system can image activity in structures as small as one-hundredth the thickness of a human hair and is fast enough to capture the changes in synaptic strength that occur on a millisecond scale. By combining this microscope with voltage sensors, the team achieved what had seemed impossible: measuring voltage signals at the level of a single synapse in the brain of a live, behaving animal.
One of the most surprising discoveries was that the synapses of the observed hippocampal neurons [located in the temporal lobe of the brain, key to learning, memory and spatial orientation] did not behave uniformly along their branched extensions, the so-called dendrites. Throughout the measurements, synaptic activity and strength varied along the dendritic branches near the apex of pyramidal cells but not near their base. “It’s still unclear why this happens or why this mechanism is important,” noted Losonczy. “We know that memories are organized at multiple levels, from synapses to individual neurons and neural circuits. Now we see that even within a single cell, there may be a level of organization.”
These findings pave the way for further studies aimed at understanding the molecular, biochemical, and genetic changes responsible for preserving the altered synaptic strength and stabilizing it at the cellular level.
This is not the first major scientific breakthrough by Losonczy’s team, achieved with the help of Hungarian developments. In 2020, their research on inhibitory neurons in the brain’s memory center was published in Neuron. This was followed in 2022 by another Nature publication demonstrating that neurons in an important memory center work together in small, coordinated networks.